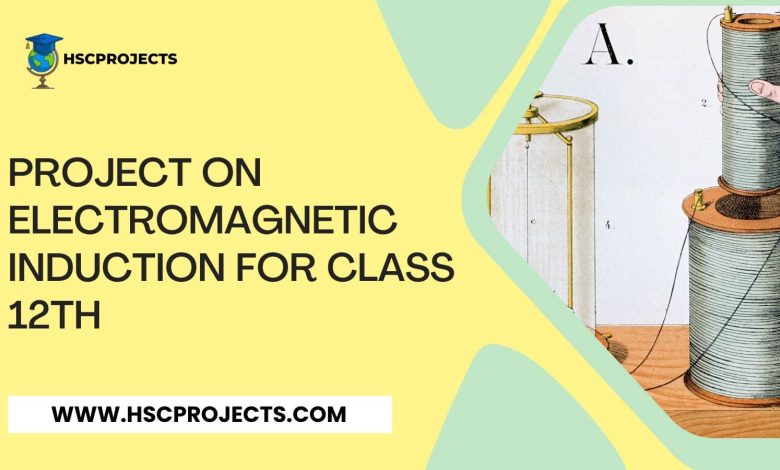
Project On Electromagnetic Induction For Class 12th
Introduction
Electromagnetic induction, a cornerstone of modern electrical engineering, is more than just a concept; it’s a force that powers our daily lives and drives our technological marvels. In this journey, we’ll unravel the essence of electromagnetic induction and its indispensable role in the world of technology.
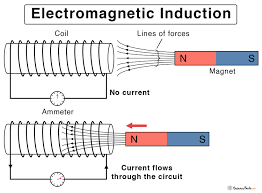
Historical Perspective
Let’s take a leap into the past, where visionaries like Michael Faraday and Heinrich Lenz laid the foundation for our understanding of electromagnetic induction. Their pioneering experiments unveiled the profound connection between electricity and magnetism.
Michael Faraday: In the early 19th century, the brilliant British scientist Michael Faraday embarked on groundbreaking experiments. His work with coils of wire and magnets revealed the extraordinary: a changing magnetic field could spark an electric current in nearby conductors. This revelation was a seismic shift, paving the way for the development of electric generators.
Heinrich Lenz: In 1834, Russian physicist Heinrich Lenz formulated Lenz’s Law. This law states that the direction of an induced current opposes the change in magnetic flux that produced it. Lenz’s Law stands as a fundamental rule in determining the direction of induced currents, ensuring that energy remains conserved within electromagnetic systems.
Principles of Electromagnetic Induction
Now, we delve into the core principles that govern electromagnetic induction. At center stage is Faraday’s Law, the beacon that illuminates how a changing magnetic field conjures an electromotive force (emf).
Faraday’s Law of Electromagnetic Induction: This law states that the electromotive force (emf) induced in a closed circuit is directly proportional to the rate of change of magnetic flux passing through it. In mathematical terms:
Where:
- emf is the electromotive force (in volts).
- N is the number of turns in the coil.
- dΦ/dt is the rate of change of magnetic flux (in webers per second, Wb/s).
This law forms the bedrock of electromagnetic induction, binding the dynamics of magnetic fields to the generation of electrical current.
Lenz’s Law
Lenz’s Law emerges as the guiding star for discerning the direction of induced currents. Through practical examples and visual demonstrations, we demystify the concept of self-consistency in electromagnetic induction.
Lenz’s Law: This principle declares that the direction of an induced current in a closed loop opposes the change in magnetic flux that gave birth to it. Lenz’s Law safeguards the conservation of energy, ensuring that electromagnetic systems abide by the laws of physics.
Imagine this scenario: Picture a coil of wire as you move a magnet closer to it. As the magnet nears the coil, it ushers in an increasing magnetic flux. Lenz’s Law steps in, dictating that an induced current will flow through the coil in a manner that counteracts the magnet’s motion. The coil acts as a magnetic brake, resisting the magnet’s advance.
Lenz’s Law is the guardian of electromagnetic induction, preserving the sanctity of energy conservation.
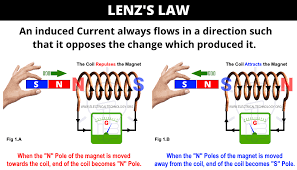
Factors Affecting Induced emf
Let’s dissect the magnitude of induced emf, diving into the factors that influence it. We’ll navigate the subtleties of magnetic flux, coil turns, and their intricate dance with induced emf.
Factors Affecting Induced emf: Several variables sway the magnitude of induced electromotive force (emf) in a conductor:
- Rate of Change of Magnetic Flux: The faster the magnetic field transforms, the greater the induced emf. This aligns seamlessly with Faraday’s Law, which links emf directly to the rate of flux change.
- Number of Turns in the Coil: An upsurge in the number of coil turns (N) augments the induced emf. Each coil turn contributes to the overall emf, making coil geometry a pivotal factor.
- Strength of the Magnetic Field: A robust magnetic field begets a more substantial emf. The relationship between magnetic field strength and induced emf follows a linear path.
- Angle Between Magnetic Field and Coil: The angle formed between the magnetic field and the coil plays a role in induced emf. Maximum emf materializes when the magnetic field aligns perpendicularly with the coil.
Comprehending these factors empowers engineers to craft electromagnetic systems tailored to precise requirements, optimizing their performance across diverse applications.
Induced Current and Electromagnetic Force
Building upon the foundation of induced emf, we unveil how it births electric currents. The right-hand rule becomes your trusty companion for determining current direction and magnitude.
Induced Current and Electromagnetic Force: When emf arises in a conductor due to a changing magnetic field, it triggers the flow of electric current within the conductor. The right-hand rule emerges as a beacon, aiding in calculating the direction and magnitude of this induced current.
Here’s the rule: If you point your thumb in the direction of the magnetic field (B), and let your fingers denote the conductor’s motion or the induced emf (V), your palm reveals the path of the induced current (I). This rule proves invaluable in predicting induced current behavior in diverse scenarios.
By wielding the right-hand rule, you gain the ability to decipher the direction of induced currents—an indispensable skill for dissecting and designing electromagnetic systems.
Self-Inductance and Inductors
Electromagnetic induction doesn’t merely bow to external forces. We introduce the concept of self-inductance and embark on a journey into the realm of inductors. They are the unsung heroes of electrical circuits, armed with practical applications and significance.
Self-Inductance and Inductors: Beyond the influence of external magnetic fields, conductors possess self-inductance. Self-inductance, symbolized as L, quantifies a conductor or a coil of wire’s capability to induce emf in itself when its current undergoes change.
Enter inductors, the purposeful components designed to harness self-inductance. Typically, they take the form of wire coils that stockpile energy within their magnetic fields as current flows through them. When this current fluctuates, the stored energy is released, ushering in emf within the coil. Inductors are veritable Swiss Army knives in electrical circuits, filtering high-frequency noise, storing energy, and timing elements in oscillators.
Familiarity with self-inductance and inductors is paramount in crafting circuits for a plethora of applications, from power supplies to radios and telecommunications devices.
Mutual Inductance and Transformers
The magic of mutual inductance takes center stage as we explore its role in transformers, the unsung heroes of electrical power distribution. Witness the metamorphosis of voltage levels.
Mutual Inductance and Transformers: Mutual inductance, an enchanting phenomenon, occurs when one coil’s magnetic field induces emf in another nearby coil. The degree of mutual inductance hinges on factors such as the number of turns in each coil, their relative positions, and the magnetic properties of the material betwixt them.
Transformers, practical devices par excellence, leverage mutual inductance to ferry electrical energy from one coil (the primary coil) to another (the secondary coil), each sporting a different number of turns. Transformers are the unsung heroes of electrical power distribution, orchestrating the transformation of voltage to levels suitable for transmission and dispersion over vast distances.
Transformers
find themselves at home in power substations, electrical appliances, and electronic gadgets—indispensable to the tapestry of modern electrical systems.
Applications of Electromagnetic Induction
This segment breathes life into electromagnetic induction through real-world applications. Generators, alternators, induction cooktops, and magnetic card readers await your scrutiny.
Applications of Electromagnetic Induction: Electromagnetic induction manifests itself in a smorgasbord of practical applications in our daily lives and industries:
- Generators: These marvels of engineering convert mechanical energy into electrical power through electromagnetic induction. Power plants rely on generators to create electricity from diverse sources—be it fossil fuels, nuclear energy, or the breezy embrace of wind and the ceaseless flow of water.
- Alternators: Close kin to generators, alternators inhabit vehicles to recharge batteries and supply electrical vigor to vehicular systems.
- Induction Cooktops: Offering culinary precision, induction cooktops exploit electromagnetic induction to heat cookware directly and efficiently.
- Magnetic Card Readers: Devices like credit card readers and keycard systems harness electromagnetic induction to decipher information stored on magnetic stripes.
These applications underscore the practical resonance of electromagnetic induction in the tapestry of modern technology and daily conveniences.
Electromagnetic Induction in Renewable Energy
Renewable energy sources usher in a new era, and electromagnetic induction is at the forefront. Peer into the workings of wind turbines and hydroelectric generators as they tap into nature’s bounty through induction.
Electromagnetic Induction in Renewable Energy: Renewable energy sources, such as the breath of the wind and the flowing embrace of water, present sustainable alternatives to fossil fuels. Electromagnetic induction plays a pivotal role in these domains:
- Wind Turbines: These graceful giants harness the kinetic energy of the wind to rotate a generator’s rotor, summoning emf and generating electricity. The principles of electromagnetic induction breathe life into the conversion of wind energy into electrical power.
- Hydroelectric Generators: Nestled within hydroelectric power plants, these generators revel in the cascade of water. Falling water sets turbines spinning, and in turn, induces emf, birthing electricity. Electromagnetic induction dances at the core of clean, renewable hydroelectric power generation.
These renewable power sources embrace electromagnetic induction to usher in a more sustainable and ecologically aware energy future.
Experiments and Demonstrations
Learning springs to life through hands-on experiments. Detailed instructions and safety measures accompany a series of captivating experiments that let you witness electromagnetic induction firsthand.
Experiments and Demonstrations: To truly grasp electromagnetic induction, nothing surpasses practical experimentation. In this section, we unveil a series of hands-on experiments and demonstrations that grant you a front-row seat to the marvels of electromagnetic induction.
Experiment 1: “Generating Electricity with a Simple Coil and Magnet” – Here, you’ll construct a basic generator using a coil of wire and a magnet. By rotating the magnet within the coil, you’ll witness the birth of emf and the emergence of electrical current.
Experiment 2: “Lenz’s Law in Action” – This experiment exemplifies Lenz’s Law, demonstrating how a moving magnet begets a current in a coil—one that opposes the magnet’s motion.
Experiment 3: “Transforming Voltage with a Miniature Transformer” – Dive into the realm of mutual inductance with a miniature transformer. Witness voltage metamorphose as you connect different coils.
Safety reigns supreme during these experiments. Always adhere to recommended safety guidelines and equip yourself with appropriate protective gear.
Conclusion
Electromagnetic induction is more than just a scientific curiosity; it’s a force that has reshaped the way we generate and harness electrical energy. From its humble origins in the experiments of Faraday and Lenz to its pervasive applications in power generation, industry, and renewable energy, electromagnetic induction continues to drive our technological advancements.
This comprehensive exploration—from fundamental principles to practical applications—serves as a beacon, guiding future generations of scientists and engineers to innovate and harness the extraordinary power of electromagnetic induction for the betterment of society and the environment.
Certificate
[Your Name] [Student ID]
This is to certify that
[Your Full Name]
has successfully completed the requirements for the course
[Course Title]
offered by
[University/Institution Name]
during the academic year
[Academic Year]
The student has demonstrated outstanding dedication, commitment, and a strong aptitude for learning throughout the course. Their performance and engagement have been exemplary, reflecting their enthusiasm for the subject matter.
This certificate is awarded in recognition of the student’s exceptional academic achievement and their valuable contributions to the learning community.
Date of Award: [Date]
Signature: _______________________________
[Professor’s Name] [Professor’s Title] [Department or School Name]
Congratulations on your outstanding achievement! We wish you continued success in your academic and personal endeavors.
[University/Institution Logo] [Seal of Approval]In order to download the PDF, You must follow on Youtube. Once done, Click on Submit
Follow On YoutubeSubscribed? Click on Confirm
Download Project On Electromagnetic Induction For Class 12th PDF
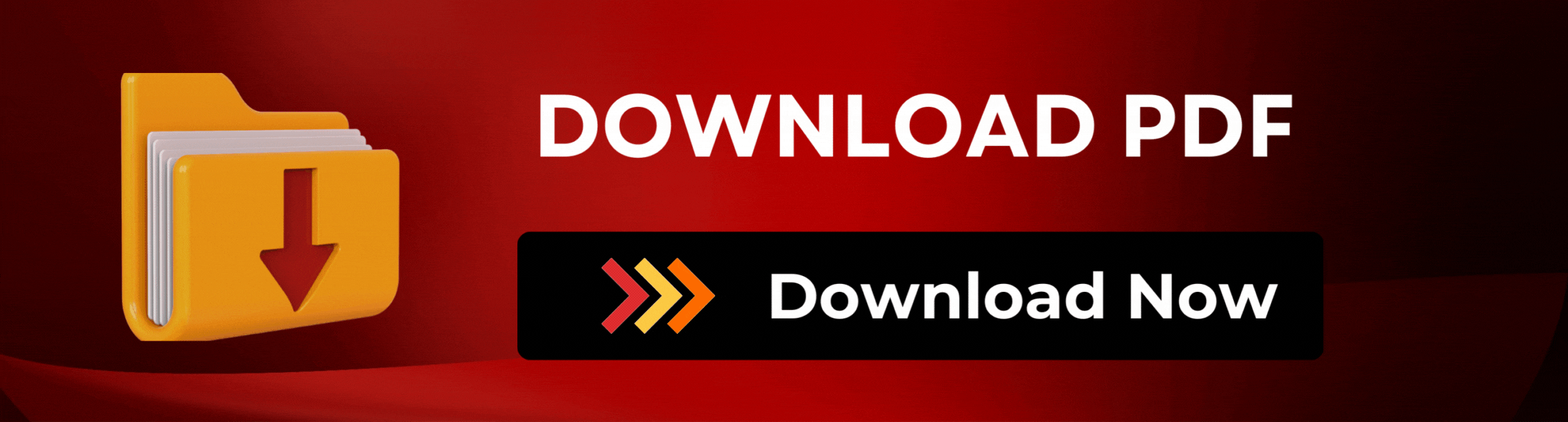