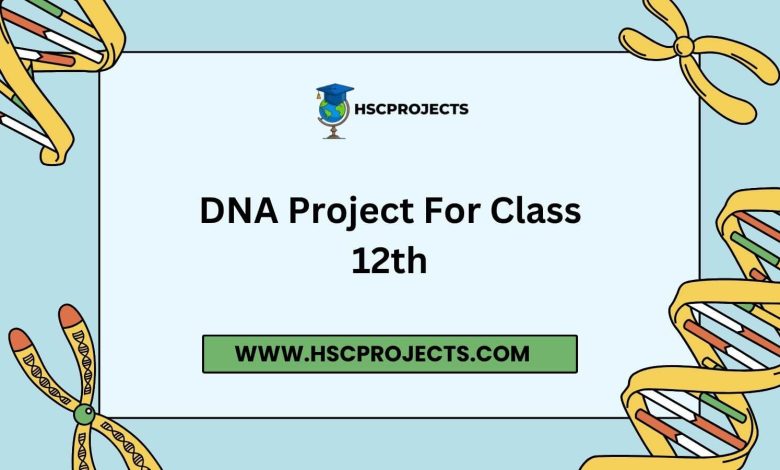
DNA Project For Class 12th
Introduction
Deoxyribonucleic Acid, commonly known as DNA, represents an intriguing acronym for a profoundly significant entity. It stands as the paramount custodian of life’s most profound mysteries. DNA, with its intricate molecular architecture and remarkable functionality, conducts the symphony of existence itself. In the captivating realm of biology, DNA earns the esteemed appellation of the “molecule of life,” and its eminence transcends all limits.
Structure of DNA
The configuration of DNA, often likened to the masterplan of life, presents an intricate and captivating molecular structure. At its core, DNA showcases an astonishing double helix arrangement, a hallmark of both its grace and functionality.
Double Helix Structure: DNA’s most distinguishing feature is its double helix structure, evoking images of a spiraling staircase or a twined ladder. This double helix comprises two lengthy and complementary strands that elegantly wind around each other. This structural elegance is pivotal to DNA’s function as it offers remarkable stability while allowing effortless access to the genetic code it harbors.
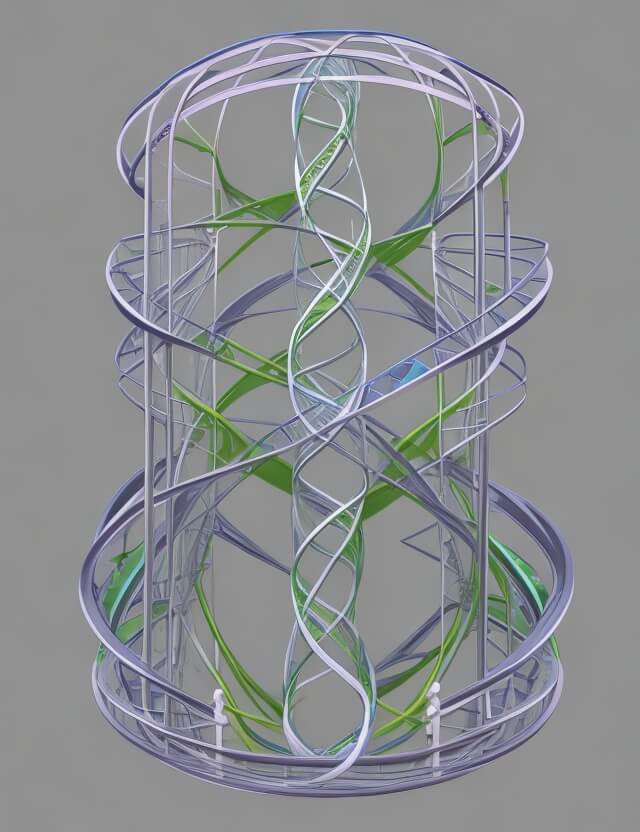
Composition of DNA Strands:
Nucleotides: The foundational units of DNA are nucleotides. Each nucleotide comprises three essential constituents: a deoxyribose sugar molecule, a phosphate group, and one of four nitrogenous bases – adenine (A), thymine (T), cytosine (C), or guanine (G). These nitrogenous bases play a pivotal role in determining the genetic information encoded within DNA.
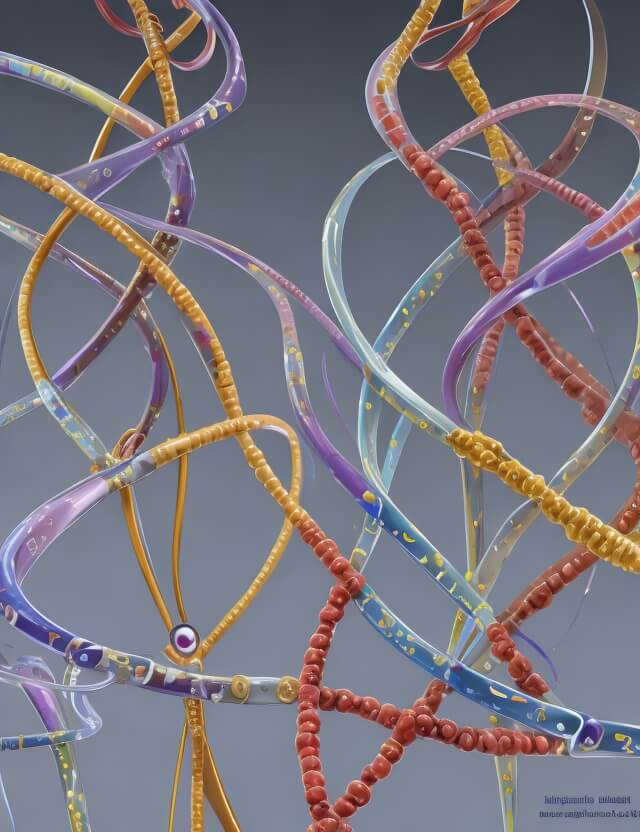
Sugar-Phosphate Backbone: Alternating with the nitrogenous bases along the DNA strands is the sugar-phosphate backbone. This backbone is akin to the sturdy handrail of the DNA ladder, providing structural support and stability to the molecule. The phosphate groups link the sugar molecules, forming robust covalent bonds that bolster the overall structural integrity of DNA.
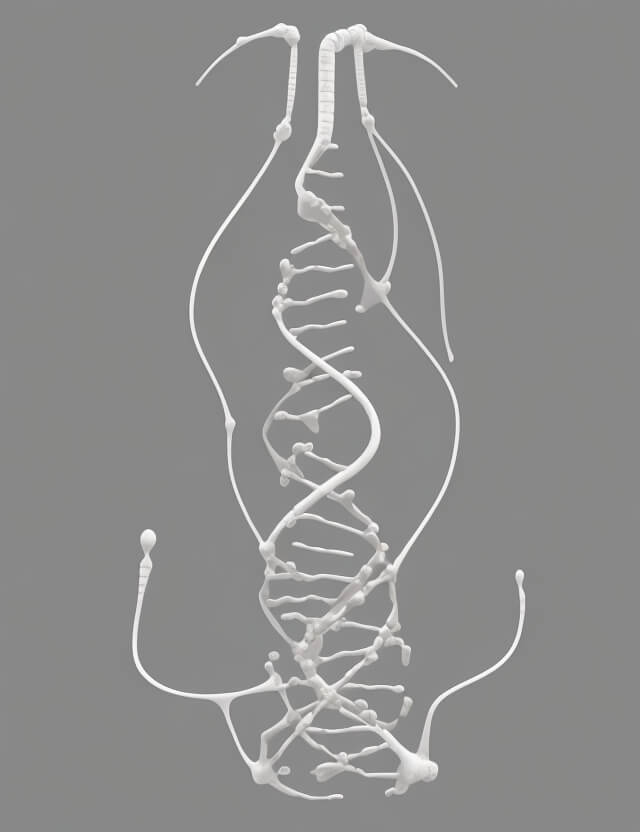
Nitrogenous Bases:
- Adenine (A): Among the quartet of nitrogenous bases found in DNA, adenine holds a prominent place. It forms complementary base pairs with thymine (T), held together by two hydrogen bonds. This A-T base pairing is indispensable for preserving the DNA double helix’s structure.
- Thymine (T): Thymine specifically pairs with adenine (A) via two hydrogen bonds. This pairing is crucial for the meticulous replication and transcription of genetic information.
- Cytosine (C): Cytosine engages in base pairs with guanine (G) through three hydrogen bonds. This C-G base pairing plays a pivotal role in upholding the stability and integrity of the DNA molecule.
- Guanine (G): Guanine pairs harmoniously with cytosine (C) through three hydrogen bonds. Similar to A-T pairing, this contributes significantly to the structural resilience of the DNA double helix.
Functions of DNA
Genetic Information Storage:
DNA takes on the role of a custodian for an organism’s genetic blueprints: One of DNA’s primary functions is to serve as the sacred vault of genetic wisdom for all living entities. Within its intricate structure lies the masterplan for constructing and upholding an organism’s architecture, functions, and unique traits.
It carries the essential blueprints for crafting proteins: DNA harbors the genetic code, a sacred sequence that dictates the arrangement of amino acids in proteins. Proteins, those essential cellular artisans, perform an array of vital duties, from lending structural support to catalyzing intricate biochemical dances. DNA’s pivotal role in orchestrating protein synthesis stands at the core of every living organism’s existence.
Heredity
DNA embodies the legacy of traits, passing them from one generation to the next: Another pivotal function of DNA is its role as the genetic baton that traverses generations. It is the sacred scroll of hereditary knowledge, passing down genetic traits from parents to progeny during the timeless act of reproduction, ensuring the continuity of the genetic saga.
Variations in DNA and Genetic Diversity:
- Genetic Diversity Emerges from the Natural Quilt of DNA: Genetic diversity, that mosaic of differences within a species, arises from the rich tapestry of DNA sequences among individuals. These variations unfold through various mechanisms, breathing life into the adaptability and evolution of species. Here, we unravel the threads of how DNA’s variations weave the fabric of genetic diversity:
- Mutation: Mutations, those subtle alterations in the DNA script, can surface spontaneously or under the influence of external forces like radiation or chemicals. These genetic brushstrokes can paint new traits, some of which may confer advantages within specific environments. With the passage of time, advantageous mutations may rise in prominence within a population, their contribution to genetic diversity akin to the strokes of an evolving masterpiece.
- Recombination: In the grand choreography of sexual reproduction, genetic recombination takes center stage. DNA from two parent entities converges, orchestrating a symphony of uniqueness in their offspring. This genetic waltz, led by processes such as meiotic crossing-over, bequeaths the progeny with a medley of traits not found in either progenitor—a genetic inheritance as intricate as a sonnet.
- Gene Flow: Gene flow, akin to the wandering minstrels of old, occurs when individuals from one population venture forth to intermingle with their counterparts from distant lands. This cultural exchange of genetic materials infuses new variations into a population’s genetic lexicon, a melodic infusion that bolsters genetic diversity.
- Natural Selection: In the theater of existence, the process of natural selection spotlights individuals endowed with traits that bestow upon them a passport to survival or reproductive success within their chosen landscapes. Over eons, these favored traits dance into prominence, their melodies echoing through generations, while less harmonious attributes may fade into obscurity. This is an eternal ballad that shapes the genetic diversity within a species.
- Genetic Drift: In the intimate gatherings of small populations, the capricious dance of chance may lead to fluctuations in the prevalence of certain gene variants, like dice tossed in a moonlit courtyard. These stochastic tides can usher in changes to genetic diversity over time, a serenade to the whims of fate in the grand genetic orchestra.
DNA Replication
Significance:
The process of DNA replication stands as a cornerstone in the realm of biology, its importance immeasurable. It serves as the lifeblood of cell division and growth, ensuring the precise inheritance of genetic wisdom from one generation of cells to the next. Without DNA replication, the grand tapestry of life would unravel, for cells would remain powerless to multiply and transmit their genetic blueprints—the very essence of life’s continuity.
The Intricacies of DNA Replication:
DNA replication is an intricate ballet, unfolding during the cell cycle, specifically in the S (synthesis) phase of interlude, before the grand spectacle of cell division (mitosis or meiosis). This meticulous choreography encompasses numerous movements, including the unwinding of the double helix, the harmonious dance of complementary base pairing, and the birth of two identical DNA twins. Here, we embark on a detailed exploration:
Initiation:
The overture of DNA replication commences at specific points on the DNA strand christened as “origins of replication.” These sacred sites bear sequences that herald the birth of replication’s symphony. Enter the virtuoso enzymes known as DNA helicases, their task—to untwist the double helix, dismantling the hydrogen bonds between the complementary base pairs, thus parting the DNA strands like theater curtains.
Complementary Base Pairing:
Each single-stranded DNA template assumes the role of a guiding maestro for the creation of a new, complementary strand. In this elegant performance, the conductor is DNA polymerase, directing the addition of nucleotides to the emerging strand in perfect harmony with the rules of base pairing: Adenine (A) forever joins hands with thymine (T) through two hydrogen bonds. Cytosine (C) waltzes with guanine (G) through three hydrogen bonds. As DNA polymerase follows the tempo of the template strand, it appends nucleotides to the nascent strand, adhering to the notes of complementary base pairs. This crescendo yields a fresh, complementary strand of DNA for each template strand.
Leading and Lagging Strands:
DNA replication unfolds as a semi-conservative masterpiece, birthing newly formed DNA molecules, each bearing one ancestral (parental) strand and one nascent (daughter) strand. Yet, the strands of DNA run in opposite directions, akin to a musical duet where harmony is achieved through discord. The leading strand dances gracefully in a continuous 5′ to 3′ direction, tracing the path of the replication fork like the lead in a waltz. The lagging strand, however, executes a dance of starts and stops. In this intricate choreography, it pirouettes in short fragments, known as Okazaki fragments, in the 3′ to 5′ direction, away from the replication fork.
Okazaki Fragment Processing:
The ballet continues with the emergence of RNA primers on the lagging strand, choreographed by an enzyme known as primase. DNA polymerase then extends these RNA primers, stringing together DNA nucleotides, forming the delicate notes of Okazaki fragments. In the final movement, DNA ligase takes center stage, uniting these fragments, stitching together a continuous strand on the lagging dancer.
Termination:
The performance reaches its crescendo at the termination site, where specialized proteins step in, raising the final curtain to halt further replication. At this zenith, two identical and complete DNA masterpieces, each born of one ancestral strand and one newly crafted strand, emerge—the testament to the artistry of DNA replication.
Genetic Code and Protein Synthesis
Genetic Code and its Connection to DNA:
The genetic code, a set of rules as intricate as a symphony, dictates how the sequence of DNA’s nucleotides (A, T, C, and G) transforms into the sequence of amino acids that form proteins. DNA stands as the parchment upon which this genetic code is inscribed. It houses the blueprints for erecting and sustaining an organism’s form and function, its secrets veiled within the sequence of its nucleotides. Think of the genetic code as a language, translating the wisdom embedded in DNA into the tongue of proteins—the tireless laborers of the cell.
Codons, tRNA, and mRNA:
Codons: The genetic code is deciphered in triplets known as codons, where each codon comprises three consecutive nucleotide bases in DNA (or RNA). These codons act as the architects, specifying either a particular amino acid or a command to commence or conclude protein synthesis.
tRNA (Transfer RNA): Enter the tRNA, the cell’s translation maestros. These adapter molecules cradle a specific amino acid and wield an anticodon region. This anticodon forms bonds with its complementary codon on mRNA during translation, ensuring that the correct amino acids join the growing protein ensemble.
mRNA (Messenger RNA): The mRNA embarks on a journey, ferrying the genetic message from DNA to the ribosome, the hallowed stage for protein synthesis. It is born from a DNA template during transcription and boasts codons that spell out the protein’s amino acid sequence.
The Ballet of Protein Synthesis:
The Process of Protein Synthesis:
Protein synthesis waltzes through two acts—transcription and translation. Transcription: In the cell’s nucleus, RNA polymerase, the conductor of this symphony, reads the DNA template strand. It then composes a complementary mRNA strand by stitching together RNA nucleotides. This mRNA becomes the courier, ferrying the genetic instructions from DNA to the ribosome. During transcription, DNA’s adenine (A) joins hands with uracil (U) in RNA, rather than its customary partner, thymine (T).
Translation: The stage for translation is set in the cytoplasm, within the ribosomes. Here, the mRNA script is converted into a unique amino acid sequence, birthing a protein. This transformation unfolds in three principal steps:
- Initiation: The overture commences as the small ribosomal subunit attaches to the mRNA at the AUG start codon. Simultaneously, the tRNA, bearing the corresponding amino acid (methionine), takes its place.
- Elongation: With the ribosome as the orchestra, tRNA instruments bring in amino acids one by one. The ribosome then joins them in a harmonious symphony, crafting an ever-expanding polypeptide chain.
- Termination: When the final note, the stop codon (UAA, UAG, or UGA), graces the mRNA script, the curtain falls on protein synthesis. The ribosome delivers the completed protein.
DNA’s Pivotal Role in Protein Synthesis:
DNA stands as the fount of genetic wisdom, and during transcription, it imparts this wisdom to mRNA. This messenger then embarks on its journey to the ribosome for translation. The DNA sequence unfurls into the complementary sequence within mRNA, assuring the faithful transmission of the genetic code from the nucleus to the cytoplasm. The genetic code in DNA is no less than the architect’s blueprint, defining the order of amino acids within a protein. This order, in turn, dictates the protein’s structure and function. Without the precise transcription and translation of the genetic code from DNA to protein, the cell would be bereft of the ability to craft the myriad proteins essential for its survival and operation.
In sum, the genetic code bridges the chasm between DNA and proteins, and through the artistry of transcription and translation, the cell brings this code to life. mRNA, tRNA, and the ribosome dance in unison to give shape to the genetic symphony, with DNA at the helm as the grand conductor of life’s magnum opus.
Applications of DNA in Biotechnology
Concept and Application in Forensics
DNA fingerprinting, a marvel of genetic science, serves as a method for distinguishing individuals based on their unique DNA profiles. In the world of forensics, DNA fingerprinting dons the cape of justice, aiding in solving crimes by matching DNA evidence collected at crime scenes (such as blood, hair, or saliva) to suspects or victims. The process unfurls with the extraction of DNA from the sample, followed by the amplification of specific regions via techniques like polymerase chain reaction (PCR). The resulting DNA fragments are then analyzed. These DNA profiles are like fingerprints of the genetic realm, and if a match is found, it can be a potent piece of evidence in a court of law.
Application in Paternity Testing:
DNA fingerprinting also plays a pivotal role in paternity testing, unraveling the mysteries of biological relationships. By juxtaposing the DNA profiles of an alleged father, child, and mother, one can ascertain whether the individual in question is indeed the biological parent of the child. This has far-reaching legal and social implications, from determining child support obligations to securing inheritance rights.
Genetic Engineering
Utilization of DNA Technology in Genetic Modification:
Genetic engineering, a realm of deliberate genetic manipulation, involves tweaking an organism’s DNA to add, remove, or modify specific genes. DNA technology is the master craftsman’s toolkit in this domain, enabling scientists to surgically extract, manipulate, and insert genes from one organism into another. This artistry gives birth to genetically modified organisms (GMOs), endowed with coveted traits—be it pest resistance in crops, elevated milk production in livestock, or the production of therapeutic proteins by bacteria.
Cloning
Concept and Applications in Agriculture and Medicine:
Cloning, akin to forging genetic twins, encompasses the art of creating genetically identical replicas of organisms or individuals. This craft boasts various techniques, including somatic cell nuclear transfer (SCNT). In the agrarian realm, cloning is the conjurer’s wand, conjuring up plants and animals with prized attributes. It breathes life into copies of high-yield crops or livestock adorned with precious traits. In the medical theater, cloning’s act holds promise in regenerative medicine and organ transplantation. SCNT, for example, births embryonic stem cells that metamorphose into diverse cell types for therapeutic pursuits. Additionally, cloning stands as a guardian of biodiversity, with potential applications in preserving endangered species by crafting clones from preserved genetic legacies.
Ethical and Social Considerations
Genetic Engineering
Unintended Consequences: Genetic tinkering carries the specter of unintended consequences, casting shadows on both the modified organism and the ecosystem. For instance, genetically modified crops may clandestinely intermingle with their wild counterparts, sowing the seeds of unforeseen ecological disruptions.
Human Germline Editing: The prospect of editing the DNA of human embryos, known as germline editing, unfurls an ethical tapestry rife with dilemmas. It weaves a tale of heritable and irrevocable effects, prompting apprehensions about “designer babies” and the revival of eugenics. The global scientific fraternity has etched guidelines that confine germline editing to medical realms, relegating non-medical pursuits to the realm of taboo.
Informed Consent: In the theater of clinical trials, where genetic therapies and treatments take center stage, the spotlight falls on informed consent. Patients must waltz with full comprehension of the risks and rewards, especially when the choreography involves experimental procedures.
Ownership of Genetic Information: The tussle over the custodianship of genetic information, particularly when deployed for research or commercial endeavors, is a contentious ethical battleground. Individuals harbor trepidations about their genetic data becoming commodities, harvested for profit without their nod of consent.
Cloning
Identity and Individuality: Cloning, especially when it contemplates humans, unfurls inquiries into the tapestry of uniqueness and individuality. Ethical quandaries encircle questions of autonomy and identity.
Health Risks: Cloning bestows upon the cloned progeny a bouquet of health risks, from genetic anomalies to premature aging, akin to the fate of some cloned animals.
Reproductive Freedom: Cloning sets the stage for debates about reproductive freedom, challenging the boundaries of whether it should be an avenue for individuals or couples to summon genetically akin progeny into existence.
The Significance of Responsible Use
The mantle of DNA technology rests heavily upon the shoulders of responsible use. It mandates the vigilant guardianship of ethical guidelines, the watchful gaze of regulatory oversight, and the unyielding transparency in research and applications. Scientists, policymakers, and the populace must assemble at the crossroads of discourse, sustaining ongoing dialogues that balance the scales of DNA technology’s benefits against its potential pitfalls and moral compass. The ethical purview must also embrace the equitable access to DNA technologies and therapies, ensuring they do not propagate the preexisting social schisms.
Societal Impact
Privacy:
The era of genetic exploration, characterized by genetic testing and personalized medicine, has thrust genetic privacy into the limelight. Genetic secrets, housed within the confines of the double helix, unveil not only an individual’s health proclivities but also revelations about their lineage and familial bonds. Genetic privacy concerns enshroud topics like data fortification, safeguarding against unsanctioned genetic data breaches, and the specter of discrimination etched in the very DNA code.
Genetic Discrimination:
Genetic discrimination, a somber societal overture, emerges when individuals face inequities or are denied opportunities based on their genetic heritage. This shadow of discrimination casts a pall over realms such as employment, insurance coverage, and healthcare access. In the theater of justice, legal scripts like the Genetic Information Nondiscrimination Act (GINA) in the United States unfurl their protective wings, seeking to shield against the scourge of genetic discrimination, be it in the realms of work or the sanctuary of health insurance.
Current Research and Advancements
Recent Research and Advancements in DNA Science
CRISPR-Cas9 Gene Editing: In the recent annals of DNA science, the star of the show has undoubtedly been CRISPR-Cas9 gene editing. This groundbreaking tool has undergone refinement, allowing for the precise and surgical modification of DNA. Its repertoire spans the correction of genetic diseases to the craft of engineering genetically altered organisms, promising applications as diverse as medicine and agriculture.
DNA Sequencing Technologies: DNA sequencing technologies have galloped forward, propelled by the likes of Next-Generation Sequencing (NGS) and Single-Molecule Sequencing. These advances have not only accelerated the pace of genomics research but have also bestowed the gift of cost-effectiveness. They have illuminated the path toward personalized medicine, genetic disease understanding, and a deeper delve into the secrets of the genetic code.
Epigenetics: In the enigmatic realm of epigenetics, the spotlight has unveiled the role of chemical embellishments to DNA, including DNA methylation and histone modifications. These chemical signatures choreograph the dance of gene regulation and have implications that ripple across the spectrum of cancer research, aging, and developmental biology.
Synthetic Biology: The canvas of synthetic biology has seen strokes of innovation, where scientists wield brushes to design and construct biological entities. The result is the birth of synthetic organisms and the creation of bioengineered marvels that span realms from medicine to industry.
DNA Nanotechnology: DNA, in a twist of fate, has become the master builder at the nanoscale. DNA origami, akin to the art of paper folding, enables the crafting of intricate nanostructures. These creations hold the promise of revolutionizing drug delivery and nanoelectronics.
Precision Medicine: The nexus of DNA sequencing and analysis has given birth to precision medicine, a paradigm shift that tailors medical interventions to the individual’s genetic tapestry. This personalized approach whispers promises of more accurate diagnostics, targeted therapies, and the elevation of treatment efficacy.
DNA-Based Data Storage: DNA, the repository of life’s code, has tantalizingly transcended its role to become a potential medium for data storage. Its dense and durable nature may one day revolutionize data archiving and preservation, taking us beyond the realm of traditional digital storage.
Potential Impact on Medicine and Biology
These advancements paint a tapestry of transformation in the fields of medicine and biology:
- CRISPR-Cas9 gene editing offers the tantalizing prospect of treating genetic disorders and preventing hereditary diseases through precise DNA editing.
- DNA sequencing technologies have ushered in an era of large-scale genomic exploration, illuminating the genetic underpinnings of diseases and revealing new avenues for drug development.
- Epigenetic research holds the key to unraveling age-related diseases and inspiring novel therapeutic strategies.
- Synthetic biology and DNA nanotechnology may birth groundbreaking solutions, from innovative biotechnology to cutting-edge materials science.
- Precision medicine is poised to revolutionize medical care, ushering in an era of personalized diagnostics and therapies.
- DNA-based data storage presents a tantalizing future for archiving and preserving information on a molecular scale.
Conclusion
In summary, this project has been a captivating voyage into the intricate realm of DNA, shedding light on several pivotal aspects:
DNA Structure: DNA unveils its elegant double helix structure, comprising nucleotides, sugar-phosphate backbones, and the quartet of nitrogenous bases (adenine, thymine, cytosine, and guanine).
DNA Functions: DNA serves as the guardian of genetic heritage, transmitting traits across generations. Variations in DNA are the architects of genetic diversity and the architects of evolutionary change.
DNA Replication: DNA replication stands as the bedrock of cell division and growth, a choreography of unwinding, complementary base pairing, and the birth of twin DNA molecules.
Genetic Code and Protein Synthesis: The genetic code orchestrates the translation of DNA’s language into the choreography of amino acid sequences in proteins. This intricate dance is conducted by codons, mRNA, and tRNA, with transcription and translation as the central acts.
Applications in Biotechnology: DNA showcases its versatility in various biotechnological realms, from solving mysteries in forensics and paternity testing to molding organisms through genetic engineering and cloning.
Ethical and Social Considerations: A tapestry of ethical quandaries envelops DNA science, spanning genetic engineering, cloning, consent, ownership of genetic information, and the clarion call for responsible technology usage. Societal echoes include privacy concerns, genetic discrimination, and the clarion call for equitable access.
Recent Research and Advancements: DNA science is not static; it’s a realm in perpetual motion. Recent strides, such as CRISPR-Cas9 gene editing, cutting-edge DNA sequencing technologies, epigenetics, synthetic biology, DNA nanotechnology, precision medicine, and DNA-based data storage, have unfurled vast horizons of possibility. They offer to reshape medicine, genetics, and biotechnology.
In this odyssey, it becomes palpable that DNA stands as the epicenter of the life sciences—a code that orchestrates the symphony of existence, the blueprint that weaves the tapestry of diversity. The research and innovations born from the study of DNA are transformative, promising to confront genetic diseases, revolutionize agriculture, and propel scientific discovery. Yet, they are also a clarion call for reflection on ethical and societal implications.
In the grand tapestry of science, DNA research is a voyage into the heart of life itself. Its discoveries and innovations resonate far beyond the confines of laboratories, shaping the destiny of medicine, genetics, and biotechnology. In the end, it influences not just science but the very essence of our existence and the world we call home.
Certificate
[Your School Logo]CERTIFICATE OF ACHIEVEMENT
I am thrilled to receive this Certificate of Achievement for successfully completing the project titled
“Understanding DNA: The Molecule of Life”
This project has been a journey of exploration, curiosity, and learning for me. I have had the opportunity to dive deep into the fascinating world of DNA, uncovering its mysteries, and understanding its pivotal role in biology. From grasping the elegant double-helix structure to unraveling the intricate processes of DNA replication and protein synthesis, this project has expanded my knowledge and ignited a profound appreciation for the “molecule of life.”
I would like to express my gratitude to my dedicated biology teacher, who guided me throughout this project, and to my fellow classmates for their support and encouragement. This project has not only enriched my understanding of DNA but also inspired a sense of wonder about the incredible complexities of life itself.
I proudly accept this certificate as a symbol of my commitment to the subject and my passion for scientific exploration. It serves as a reminder of the incredible journey I’ve embarked on and the endless possibilities that await in the world of biology.
Awarded this [Date] day of [Month], [Year].
[Student’s Full Name][Class/Grade: 12][School Name]
In order to download the PDF, You must follow on Youtube. Once done, Click on Submit
Follow On YoutubeSubscribed? Click on Confirm
Download DNA Project For Class 12th PDF
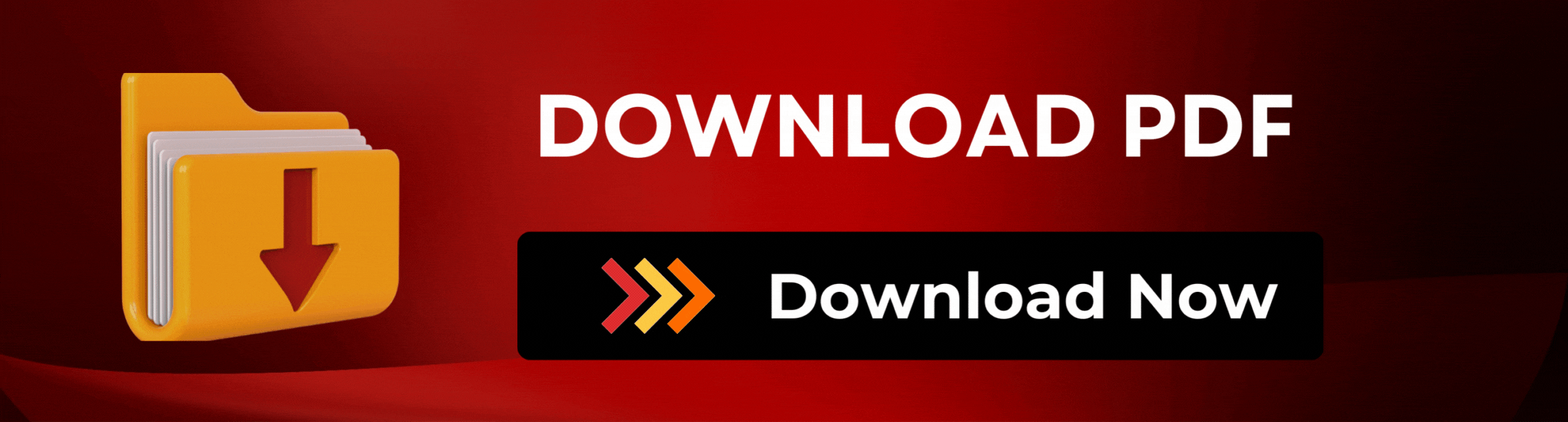